Scientist II, Formulations, Biologics
Biologics have unique developmental challenges on their pathway to becoming a commercial product. Today’s biologics are developed utilizing a wide variety of modalities, including antibodies, peptides, enzymes, fusion proteins, antibody-drug conjugates, gene therapies and cellular therapies (e.g. CAR-Ts). While this breadth of modalities has opened up biologic development to new possibilities, it has also required innovation to overcome developmental hurdles. In biologics development, one of the most promising innovations is engineered micro- or nano-particle technology.
The US Food and Drug Administration (FDA) states that nano-technology can add new aspects to newly-developed or existing biologics, as follows: “The application of nano-technology may result in product attributes that differ from those of conventionally-manufactured products, and thus evaluations of safety or effectiveness of FDA-regulated products that include nanomaterials or otherwise involve the application of nanotechnology should consider the unique properties and behaviors that nanomaterials may exhibit.”1-3 Indeed, nano-technology has the potential to add unexpected benefits to existing, or newly-developed, biologics.
One notable example of incorporating nano-technology during biologic development is Halozyme Therapeutic’s ENHANZE® drug delivery technology, approved by the FDA in 2018. With ENHANZE®, traditional intravenously-administered (IV) biologics may instead be subcutaneously (SQ) injected, which reduces administration time, injection pain, and infusion site reactions. Typically, SQ injections are only available up to a few milliliters of volume per injection, so current efforts are focused on developing high concentration protein formulations in the fixed volume.
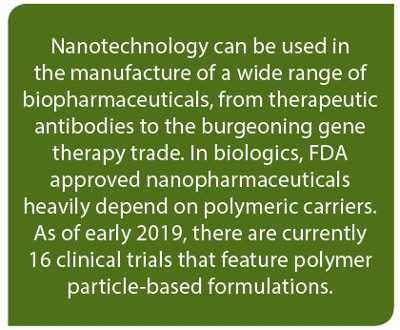
The rHuPH20 enzyme (Halozyme’s HYLENEX®) raises the bar for SQ injection, and opens the door to self-administration of a much higher volume, combined with a large volume self-injector device. It works for SQ fluid administration by leveraging hydration to increase the dispersion and absorption of other injected drugs. The co-administered therapeutics degrades the glycosaminoglycan hyaluronan (HA) and enables the main drug molecules, such as an antibody, to be dispersed more evenly. Using this method, a self-administrated SQ injection can replace a costly, painful IV infusion route.4
In the last two decades more than 50 nano-pharmaceuticals have received FDA approval and are available for clinical use.3 Dosage delivery is typically in oral, IV or transdermal administration. Among approved nano-pharmaceuticals, polymer, micelle, liposome, and nanocrystal forms comprise most of the nano-drugs, but a few metallic and protein particles are present as well. Nanopharmaceutical are becoming the platforms for a broad range of complex nano-particle types.
Nanotechnology can be used in the manufacture of a wide range of biopharmaceuticals, from therapeutic antibodies to the burgeoning gene therapy trade. In biologics, FDA approved nanopharmaceuticals heavily depend on polymeric carriers. As of early 2019, there are currently 16 clinical trials that feature polymer particle-based formulations (clinicaltrials.gov).
Polymeric Carriers
When utilizing polymeric carriers with biologics, expected benefits include: improved circulation time in case of intravenous injection; decreased immunogenicity or lower systemic toxicity; controlled, sustained release; improved stability; targeting effect to desired local delivery; improved solubility; increased drug dosing and bioavailability or faster administration at higher dose.
Dependent on the intended purpose, the delivery form of a biologic is designed as a systemic injection or local delivery, and should be considered when designing the particle delivery system. So far, FDA approved, polymer-based particles are mostly intended for greater protein solubility, longer circulation time, longer half-life and controlled release to reduce the number of injections. In a few cases, protein nano-particles are designed based on the carrier system for better efficacy or targeting.3 Polymer-based particles are typically favored for biologics because they have the flexibility and availability to direct conjugation to proteins, which is required for the large payload needed to encapsulate large molecules.
PLA/PLGA-Based Drugs
Currently 15 FDA-approved polylactide (PLA)/polylactide-co-glycolide (PLGA)-based drug products are available on the US market. PLA/PLGA is a family of FDA-approved biodegradable polymers that are stable enough and greatly biocompatible, they have been comprehensively studied as a delivery vehicle for small molecules, proteins and various other macromolecules such as DNA, RNA and peptides. Because of its long, proven clinical existence, favorable degradation features, and prospects for sustained drug delivery, PLA/PLGA has been the most prevalent among the various available biodegradable polymers. Recent literature has shown that degradation of the PLGA delivery system can be adapted for sustained drug release at desirable doses without surgical procedures.2
As a rule of thumb, particle degradation and drug release rate can be controlled by size, hydrophilicity and crystallinity. All these factors should be considered in order to adjust the degradation and drug release mechanism for the desired application. For example, for very long-term release, more than half year, semi-crystalline or high crystallinity polymers can be considered. Research reports that PLGA can easily be adapted to encapsulate a wide range of biologics products such as peptides, proteins and nucleotides, by desired periods of time with diverse routes of delivery.
There are many different types of polymeric nanocarriers. Early studies in this area mainly use bio-compatible polymer, such as PLGA, to form NPs through different oil-in-water emulsion techniques. Polymeric micelles have also been extensively used for oligonucleotide delivery strategies.5 These are made by self-assembly of amphiphilic polymers in an aqueous environment. A third type of polymeric carrier is the hydrogel.6 These systems have an open, water-filled polymer lattice that can easily incorporate bio-macromolecules such as protein and oligonucleotide, whose release kinetics can be controlled by the degree of cross-linking of the polymer matrix.
Monoclonal Antibodies
Monoclonal antibodies (mAbs) are the current major product class in biologics. To increase their efficacy, researchers are working to extend their effectiveness and circulation lifespan. One example of these next-gen mAbs is Cimzia®, the FDA-approved Certolizumab pegol, a PEGylated (polyethylene glycol) anti-TNFα:7 the product is a humanized antigen-binding fragment (Fab’) of a mAb that has been conjugated to PEG.
And mAb technologies are continuing to evolve to develop medicines with increasingly improved safety profiles, such as nanobody technology.8 The development of long acting protein formulations must uphold protein stability and be able to deliver enough doses over a prolonged period. Developmental scientists are trying to improve the formulation and dosage forms of antibodies to improve efficacy and to increase the range of applications.9
Gene Therapies
Gene therapy is primarily available in a research and development setting. The first gene therapy product was approved in 2017 by the FDA10 and there have only been a few other gene therapy products approved in the United States since then.11 That could change soon, though. Literally hundreds of research studies (clinical trials) are underway testing gene therapy as a treatment for rare diseases, cancer, and HIV/AIDS.12 Two recently approved gene therapy drugs used T cells extracted from blood cancer patients and then genetically altered the T cells to produce surface receptors, called chimeric antigen receptors (CAR-T), that seek out and kill cancer. The FDA also approved the first direct injection form of an adeno-associated viral vector gene therapy product last year.
Disruptive technologies, such as next generation DNA sequencing (NGS), has switched on genomics to identify new disease targets, and those targets are easily fixed by gene therapy strategies. Future therapeutics will be more prevailing with nucleic acid-(siRNA, mRNA, or aptamer) based therapies or small peptides, due to easy of production and targeting. But their cellular entity presents challenges, mainly due to the charged properties of nucleic acids and proteins. Enhanced drug delivery is expected to solve this issue for the next generation of therapeutics.5,6,13
Scale-Up and Commercialization
Scale-up for commercialization of NPs has been a challenge, because conventional NP fabrication techniques are likely to have polydispersity and batch-to-batch variations. For instance, the size of NPs generated by emulsion techniques is governed by the size of the emulsion droplets, and it is intrinsically heterogeneous in bulk mixing.14 This heterogeneity remains a big obstacle in commercial scale DS (drug substance) preparation. Microfluidics can manipulate fluid consistently in nano- or pico-liter scale and it can provide good solution for the fabrication and can be extended to actual manufacturing. Microfluidics can generate a homogeneous reaction environment, enhanced processing accuracy and efficiency, a miniaturized setup, and cost savings from reduced consumption of reagents.13,15
Conclusion
The development space utilizing nano-technology has been significantly expanded and advanced during the past decade. In addition to the products that have received FDA approval, many more are being developed or are in clinical trials. The improved efficacy of antibody biologics and proof-of-concept in gene therapy pushes the needs for advanced drug delivery strategy. The amount of work that is occurring in this field predicts that new technologies will eventually be available for clinical use. While we still face many challenges for development, it may only be a matter of time until proposed strategies provide better or unique solutions for patient’s clinical needs.
References
- FDA’s Approach to Regulation of Nanotechnology Products. 2018; Available from: https://www.fda.gov/scienceresearch/specialtopics/nanotechnology/ucm301114.htm.
- Hirenkumar K. Makadia, S.J.S., Poly Lactic-co-Glycolic Acid (PLGA) as Biodegradable Controlled Drug Delivery Carrier. Polymers, 2011. 3(3): p. 1377-1397.
- Ventola, C.L., Progress in Nanomedicine: Approved and Investigational Nanodrugs. P&T, 2017. 42(12): p. 742-755.
- Locke KW, M.D., LaBarre MJ, ENHANZE® drug delivery technology: a novel approach to subcutaneous administration using recombinant human hyaluronidase PH20. Drug Delivery, 2019. 26(1): p. 98-106.
- Kazunori Kataoka, A.H., Yukio Nagasaki, Block copolymer micelles for drug delivery: Design, characterization and biological significance. Advanced Drug Delivery Reviews, 2012. 64: p. 37-48.
- J.Zachary Hilta, M.E.B., Configurational biomimesis in drug delivery: molecular imprinting of biologically significant molecules. Advanced Drug Delivery Reviews, 2004. 56(11): p. 1599-1620.
- Niti Goel, S.S., Certolizumab pegol. mAbs, 2010. 2(2): p. 137-147.
- Muyldermans, S., Exploiting Nanobodies’ Singular Traits. Annual Review of Biochemistry, 2013. 82: p. 775-797.
- Yanan Cui, P.C., Binlong Chen, Suxin Li, Hua Guan, Monoclonal antibodies: formulations of marketed products and recent advances in novel delivery system. Drug Development and Industrial Pharmacy, 2017. 43(4): p. 519-530.
- FDA. Approved Cellular and Gene Therapy Products. 2019 [cited 2019].
- Stephanie Tran, P.-J.D., Brandon Piel, and Prakash Rai, Cancer nanomedicine: a review of recent success in drug delivery. Clinical and Translational Medicine, 2017. 6(44): p. 1-21.
- Zhang B., C.G., Palczewski K., Lewis K., Ho J, FDA Approves First Gene Therapy in the United States. Rare Disease Review, 2018.
- Ying Zhang, H.F.C., Kam W. Leong, Advanced materials and processing for drug delivery: The past and the future. Advanced Drug Delivery Reviews, 2013. 65: p. 104-120.
- Salem, V.B.J.M.G.K., Biodegradable Particles as Vaccine Delivery Systems: Size Matters. The AAPS Journal, 2013. 15(1): p. 85-94.
- Qiaobing Xu, M.H., Tram T. Dang, Todd Hoare, Daniel S. Kohane, George M. Whitesides, Robert Langer, Daniel G. Anderson, Preparation of Monodisperse Biodegradable Polymer Microparticles Using a Microfluidic Flow‐Focusing Device for Controlled Drug Delivery. Small, 2009. 5(13): p. 1575-1581.