In experiments that rely on rodents as research models, the control and monitoring of both genetics and health status are key steps to mitigate variability. Current research demonstrates that the gut microbiome is a significant and overlooked variable that has only recently been considered in rodent models. Specifically, the research community has found that therapeutic response can be directly linked to the health of a model’s gut microbiome.
The use of research models with a defined and consistent gut microbiome could eliminate this newly defined variable. However, access to models with a defined static background is a challenge for life science researchers. Preventing microbial drift once the defined static background is established complicates this strategy. Avenues for standardizing and maintaining the microbiome are possible, but may take tremendous efforts and resources. This article will discuss the recently recognized impact of the gut microbiome on research and the difficulties and possibilities for controlling and monitoring this newly found variable in our rodent models.
Background
The laboratory mouse model has gone through considerable changes and refinement in the last 100 years. To remove variation in experimentation, inbred and outbred mouse strains were developed so that researchers around the world could work with identical genetics across institutions. Towards the end of the last century, the advent of genetically modified research models led to mouse models that better mimicked human disease, and they have since become the foundation for basic research and new drug development.
In recent years, the environment and husbandry of laboratory mice has also been vastly improved by using IVC racks, standardized food, bedding and water, and both biosecurity and animal welfare programs.
At a substantial cost, we can also now use genetic monitoring, phenotyping, and infectious disease screening to ensure that mouse models meet certain specifications. And, just when vivarium managers think they have successfully integrated the best conditions for their colonies, comes a new wrench - the microbiome. We now have a thorough awareness of how the mouse microbiome is impacting experiment outcomes and therapeutic efficacy.
It is not just that the bacteria in the gut of research mice may influence results, it’s that the presence or absence of specific bacteria may directly determine if a therapeutic works or not.
Microbiome and Cancer
Two very clear examples can be found in cancer research. The first involves cyclophosphamide, a clinically important cancer drug capable of waking up the cancer fighting cells in the body.1 One study reported that tumor-bearing mice that were either germ-free or given antibiotics to kill Gram+ bacteria were resistant to cyclophosphane treatments and had a reduction in T helper cell response. Adoptive transfer of the T helper cells partially restored the anti-tumor efficacy of cyclophosphamide. It was determined that the action of the cyclophosphamide alters the composition of the microbiome in the small intestine and promotes the translocation of select Gram+ bacteria across the mucosal barrier and into secondary lymph tissue. It is the presence of these bacteria in these tissues that stimulates T helper and memory Th1 immune responses needed to attack the cancer.
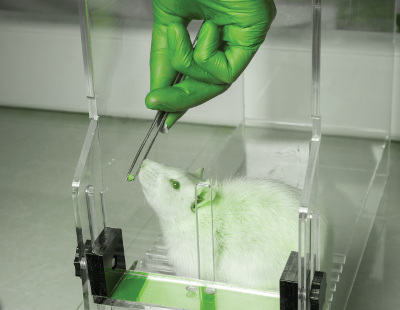
The second anti-cancer therapeutic is gemcitabine, used to treat pancreatic, lung, breast and bladder cancers.2 Resistance to this drug was observed in tumor-associated fibroblasts that secreted a hepatocyte growth factor. A logical progression of experiments and observations led to the discovery that gammaproteobacterial produce a bacterial enzyme capable of metabolizing gemcitabine. Tumor bearing mice were inoculated with E. coli, with and without antibiotic treatment. Antibiotic treated mice displayed a marked anti-tumor response to gemcitabine, whereas mice that did not receive antibiotics presented with rapid tumor progression. No gemcitabine resistance was observed when E. coli with a deficiency in the gemcitabinemetabolizing enzyme was inoculated into tumor-bearing mice and treated with gemcitabine.
These examples demonstrate the impact of the gut microbiome on two cancer therapies, but for one treatment the presence of a group of bacteria was required for a positive therapeutic response, whereas for another therapeutic, the absence of a group of bacteria was required for a positive response. And these are only two of the many examples of the microbiome influence on cancer therapeutic performance.
What does this mean for our cancer drug pipeline or other disease therapeutics that may be impacted by the gut microbiome? If a positive response is dependent on the constituents of the gut microbiome, could potential drugs be wrongly disregarded early on at the basic research level if a mouse model with a confounding microbiome is used? Alternatively, a drug with a successful outcome in a research mouse could produce a poor response in the human clinical trial if the necessary flora for a positive outcome is not present in the human subjects.
Manufacturing Microbiomes
There are other challenges as well, including the multiple vendors supplying research animals. Vendors have been very accommodating to the lab animal community by providing routine screening of the genetics and infectious agents for their standard strains and making course corrections when the genetics or health status has been compromised. However, when it comes to the microbiome, vendors are unable to provide large quantities of standard strains with the promise of a defined and stable gut microbiome.
This variation in the microbiome among vendors has not gone unnoticed by the research community. Mouse models are used to emulate human infections for many pathogens, and the response by mice to pathogen challenges have been directly associated with differences in the microbiomes among vendors. Researchers working with plasmodium, the etiological agent responsible for malaria, observed that genetically similar mice obtained from multiple research vendors displayed different severities of infection when challenged with the organism.3
Germ-free mice, which lack the presence of bacteria, were inoculated with fecal content transplants from “resistant” or “susceptible” vendor mice and presented with the respective phenotype when infected with plasmodium. Antibiotic treatment followed with consumption of a yogurt containing Lactobacillus and Bifidobacterium, both found in large amounts in resistant mice, had a decreased parasite burden.
Researchers working with Salmonella also observed differences in susceptibility among genetically similar mice from different vendors.4 Similarly, the use of germ-free mice inoculated with fecal material from resistant and susceptible mice, confirmed that the phenotype was associated with the gut microbiome, not the mouse genetics. Additional investigation identified Enterobacteriaceae, a low abundance taxon, as the keystone bacteria.
Microbiome Drift
The obvious path forward might be for laboratory mouse vendors to develop and stabilize standard microbiomes: a prospect much more complicated than it sounds. Unfortunately, a substantial number of influences have been identified that can contribute to “microbial drift” within a colony of mice, which is reviewed in detail by Ericsson and Franklin.5 Not surprisingly, food is on the list, but the manufacturer, formulation, and method of decontamination are sub-variables that can independently contribute.
Bedding material, water treatment, method of birth, as well as housing and other environmental conditions, can also influence the microbiome. To say that there is a specific vendor flora would not be accurate since most vendors have multiple barrier rooms, isolators, and multiple housing schemes that house colonies of standard strains around the world. Among these different housing types are different levels of accepted biosecurity, most being specific pathogen free (SPF), but some permitting opportunistic pathogens.
Most mice that are shipped from cages or isolators must traverse transfer hoods that could expose the mice to the flora of laboratory animal technicians as the air vortexes in and out of the transfer cabinet. Some vendors produce cleaner mouse strains in individually ventilated cages (IVC), which may create variation in the microbiome within the colony if some obligate anaerobes are not successfully associated with offspring. The use of IVC caging can create sub-populations of mice with slight microbiome variation from cage to cage.
A change of animal technicians, source of food nutrients, or the introduction of new strains into a barrier, all have the potential to contribute to microbial drift within a colony. For rodent vendors, the introduction of a single excluded pathogen could drive the complete depopulation of an entire production room, so researchers would be forced to obtain new standard strains from another room or vendor, along with a different gut microbiome.
There is likely to be differences not only among vendors, but within vendor facilities, barrier rooms and cages, and there cannot be expectations that the microbiome of vendor mice will be stable and static from one order to the next. However, the more elements you can keep constant between colonies, the less likely you will observe drift.
If you remove the above influences that contribute to change, you still need to prevent new microbes from being introduced into a colony if you want to stabilize the microbiome. The same methods commonly used for germ-free mice can be used to maintain a static flora, but the success in doing this is equal to the effort that must be exerted. It is generally agreed that germ-free mice must be maintained in sealed isolators under positive-pressure HEPA-filtered air and all husbandry materials must be decontaminated within an autoclaved canister that is ported through a gas- or decontaminant-sterilized entry port into the isolator.6 Materials have improved, as well as quality control measures, so although it is still complicated to produce and maintain germ-free mice, the known factors for success are well understood.
The limitation to these efforts is the cost of associated labor and materials, which makes this effort very expensive. Using this system for studies creates an additional challenge, since studies that involve changing or influencing the microbiome cannot be performed in the production isolator. Some institutions will produce mice within isolators, but remove mice onto standard IVC racks for short term studies (1-2 months) with the understanding that microbes may be introduced.
There has been some anecdotal evidence that suggests hermetically sealed IVC racks, in combination with careful changing station procedures, can prevent contamination for several months or years. It may be possible to use less labor-intensive methods as long as the end product is zero exposure to a contaminating organism. At one of our rodent production sites, we have been able to maintain for 13 months a stable complex microbiome at the species level, within two separate isolators, without the use of an autoclave canister.7 Instead, surface decontamination of autoclaved packaging in the entry port has been used to protect the isolator from contamination. Complexity may not be as important as careful husbandry, and processes to make sure husbandry materials and transport vessels are properly decontaminated prior to entry, may be at the base of effectiveness.
Challenges of Microbial Flora
Another challenge for researchers is to determine what microbial flora should be used for their particular study. Germ-free mice serve as a blank slate for building a custom consortium of whatever bacteria researchers wish to use, as long as the mouse can be colonized. As an alternative to germ-free mice, antibiotic treatment can also be used to clear the path for a new gut microbiome, but elimination of certain populations, including resistant bacteria is usually not complete, and the physiology of the mouse development may have already been set by the pre-existing flora.8
Even when working with germ-free mice, after adding a new bacteria consortium, the mouse will continue to have its physiological development based on a germ-free mouse. A comparison of germ-free and conventional SPF mice of the same strain found that in germ free mice, both white and grey matter were underdeveloped and the mice underperformed in standard behavioral tests compared to mice with the SPF flora.9 For this reason, it is the offspring of the associated germfree mice given the new associated flora that will have developed a physiology based on the new flora.
Some argue that a third generation may be needed, especially in the case of behavioral studies, since the offspring of the second generation will have been raised and influenced by the behavioral attributes of the associated germ-free mouse or other epigenetic contributions. Maintaining a base colony of mice colonized with a minimal microbiome or “Scaffolding flora” is another approach to having a “more normal” mouse physiology, to which one or more bacteria or none can be added to serve as a basic and static microbiome model. The altered Schaedler flora (8 bacteria members) and the oligo mouse microbiome (12 bacteria members) are older and more recent examples of scaffolding flora, with the later having the advantage of having colony resistance to the infection of pathogenic bacteria.10,11
On the extreme side, immunologists now recognize that the common SPF laboratory mouse and rat flora offer an untrained and undeveloped immune system equivalent to the immune system of a neonatal human, and that the wild mouse flora provides all the stimulation needed to produce a model that has an immune system which more closely resembles an adult human.12
Static microbiome colony monitoring requires 16S ribosomal RNA next generation sequencing, which is used to determine the relative abundance of the bacteria from which any major shifts in the microbial constituents can be identified. Although bacteria are the focus when monitoring the gut microbiome, routine health monitoring of colony mice should also include monitoring for viruses, fungi, and parasites.
Conclusion
As the influence of the microbiome on research becomes more recognized, lab animal vivariums will need to work with researchers to identify the best means to provide and maintain research models that meet their needs. Researchers will need to determine their need for a stable and consistent gut microbiome and potential advantages of this additional variable to consider in their development of a research model.
References
- Viaud, S., et. al., The intestinal microbiota modulates the anticancer immune effects of cyclophosphamide. Science Nov 22, 342(6161): 971-976, 2003.
- Geller L.T., et. al., Potential role of intratumor bacteria in mediating tumor resistance. Science Sep 15, 357(6356): 1156-1160, 2017.
- Villarion, F. et. al., Composition of the gut microbiota modulates the severity of malaria. PNAS Feb 23 113:8 2235-2240, 2016.
- Valazquez, E.M. et. al., Endogenous Enterobacteriaceae underlie variation in susceptibility to Salmonella infection. Nature Microbiology Jun 4(6):1057-1064, 2019
- Ericsson and Franklin, Microbiota and reproducibility of rodent models. Lab Animal, 46(4): 114‐122. 2017
- Nicklas, W., Maintaining and Monitoring the Defined Microbiota Status of Gnotobiotic Rodents. ILAR Journal, 56:2 241–249, 2015
- Berard, et. al., Maintaining a static microbiome across your research projects: Can it be done? Abstracts of 14th FELASA congress 2019, Maintaining a static microbiome across your research projects: Can it be done? Laboratory Animals, Vol. 53 117-118, 2019
- Kennedy E. A. et. al., Mouse Microbiota Models: Comparing Germ-Free Mice and Antibiotics Treatment as Tools for Modifying Gut Bacteria. Frontiers in Physiology, October 2018 vol. 9 | Article 1534
- Lu, J., et. al., Microbiota influence the development of the brain and behaviors in C57BL/6J mice, PLoS ONE 13(8): e0201829. 2018.
- Brand, M. W., et. al., The Altered Schaedler Flora: Continued Applications of a Defined Murine Microbial Community ILAR Journal, 2015, Vol. 56, No. 2, 169–178
- Brugiroux, S., et. al., Genome-guided design of a defined mouse microbiota that confers colonization resistance against Salmonella enterica serovar Typhimurium Nat Microbiol. 2016 Nov 21 2:16215.
- Beura, L.K., et. al., Normalizing the environment recapitulates adult human immune traits in laboratory mice. Nature volume 532, pages 512–516 (28 April 2016)