Abstract
Novel drug delivery systems offer impactful solutions to improve upon existing pharmaceutical products. These delivery systems often lead to enhanced bioavailability as well as improved systemic and local safety profiles. In addition, assessing alternative delivery methods for existing drugs or biosimilars can benefit from efficient regulatory approval.
Here we discuss the importance of preclinical models in the evaluation of novel drug delivery systems, including toxicology, pharmacokinetics and dynamics, and patient considerations. The correct pharmaceutical assays must be carefully considered in order to help inform decision making in drug development from candidate selection to preparation for clinical trial. We highlight the need to match preclinical development strategy with the right model systems, from in vitro cell monolayers to in vivo animal models of specific disease states. Novel ocular delivery systems are discussed in this context.
Benefits of Novel Drug Delivery Systems
There are great costs involved in taking new pharmaceutical products to market. Developing Novel Drug Delivery Systems (NDDS) for existing products offers an alternative approach to improving therapeutic action. A drug delivery system is the method for administering therapeutic agents to their target site.1
Streamlined approval for 505(b)(2)
Gaining regulatory approval for a novel drug delivery system of an existing, FDA-approved product can benefit greatly from fast-tracked approval. Traditional new drug applications (NDAs) that fall under the 505(b)(1) procedure require full safety and efficacy reports to be conducted solely by the applicant.2 In contrast, novel drug delivery systems are able to be approved via the 505(b)(2) route. The major benefit to this is the inclusion of safety or efficacy information not generated by or for the applicant. Thus, NDAs for new drug delivery systems can ‘piggy-back’ off data from original 505(b)(1) applications or published work to streamline the application process.
Improved patient compliance
Patient non-compliance represents a serious barrier to therapeutic efficacy, and was estimated to cost the US $528.4 billion in 2016.3 As such, it represents a major consideration in drug development. Though intravenous injection is a popular route of administration (ROA) due to its fast-acting and reliable pharmacokinetic profile, injection routes have significant barriers to patient compliance and satisfaction. Oral and intra-oral administration routes, such as sublingual (under tongue) or buccal (between the gums and cheek), offer improved compliance but place additional barriers to bioavailability (e.g. poor absorption, rapid first-pass metabolism).4 New formulations can help overcome these barriers, improving patient compliance whilst maintaining pharmacological efficiency.
Decreased administration frequency
Frequency of administration is a major factor in patient non-compliance. Route of administration and improved drug formulation can help reduce dosing frequency, through altering the pharmacokinetics of the active compounds, leading to slow-release, long-acting drugs.
Improve safety profile
Many drugs come with undesired side effects. Understanding where these come from and acting to minimize their impact goes a long way to streamlining regulatory success and improving patient satisfaction. Side effects can arise from formulation components, interaction between active compound and ROA-specific tissues (e.g. skin irritation) and toxins generated from drug metabolism. Nanomedicine delivery systems (e.g. liposomes) have seen success in lowering toxicity and enhancing site-specific delivery, such as liposome-mediated delivery of Irinotecan for treatment of colon cancer.5
Improved PK-PD profiles
As mentioned, different ROAs have broadly different pharmacokineticpharmacodynamic (PK-PD) profiles that will determine the range of pharmacological profiles any specific drug will fall within. However, a better understanding of the nature of drug fate within the ROA can lead to dramatic improvements in profiles within this range.
Challenges of Novel Drug Delivery Systems
When developing NDDS, it is important to appreciate the breadth of ramifications for drug action a new delivery system can have. As discussed above, NDDS can alter the toxicology, tolerability, pharmacokinetics and dynamics of a drug. Whilst this can be leveraged to improve drugs, it can also lead to unexpected changes in drug pharmacology. In particular, drug formulations often require optimization for a specific route of administration and moving to a new delivery system may require re-testing and candidate selection for improved formulations. Figure 1 shows a comparison of bioavailability from oral and sublingual dosing of a drug, conducted by Absorption Systems. In contrast to general assumptions about these ROAs, sublingual administration had ~11% reduced bioavailability compared to oral, due to the lack of drug formulation optimization.
Current Models of Oral Drug Bioavailability
Oral administration represents the most common ROA for pharmaceuticals.6 Solid formulations have high levels of drug stability and dosage accuracy, as well as being cheap to produce. However, the bioavailability of orally administered drugs vary significantly based on factors including: solubility, stability in the GI tract, absorption by intestinal epithelia, first-pass metabolism and reabsorption rates.7 Thus, there is a need for models to assess the pharmacokinetics of orally administered drugs as a proxy for in vivo bioavailability.
Caco-2 monolayers are a simple model of the intestinal epithelial cell layer derived from a human colon carcinoma line.8 They are primarily used for assessing bi-directional absorption/reabsorption across the monolayer, and efflux mechanisms interrogated using P-gp and BCRP inhibitors. The system can be refined to assess effects of altered intestinal fluid properties (e.g. pH). Absorption Systems has used Caco-2 monolayers to further evaluate impact of food viscosity on dissolution and permeation of the active pharmaceutical ingredient (API).9
Excised tissue assays have physiological tissue architecture and retain many physiological parameters (pH, enzymes, mucus layer).10 Ex vivo skin and corneal models have also become a popular means of assessing kinetics of both dermal and ocular topically applied drugs, where they have been used by Absorption Systems to evaluate drug accumulation in specific corneal and skin layers and skin dryness.
Intestinal perfusion is an in situ assay using an anaesthetized animal model, typically rat, to assess drug uptake along defined sections of the GI tract. The system facilitates many of the same end points of drug absorption, transporter-specific uptake/efflux, and formulation efficacies of simpler systems in a physiological context.
Benefits of in vivo Systems
In vivo models remain the gold standard for assessing safety and bioavailability for NDDS, and are a prerequisite for understanding how a drug functions in a living organism prior to clinical trials.
In vivo models provide the most accurate assessment of drug pharmacokinetics and incorporate processes such as first-pass metabolism by the liver, drug-drug interactions and drug excretion.11 Whilst in vitro toxicology screens are useful in candidate selection stages of drug discovery, proper assessment of toxicology, tolerability and dosing regimens require fully intact biological systems.1 Another major advantage of in vivo systems is the accurate representation of organism-level states that have important implications for drug function. In vivo models of disease states can incorporate systemic changes that alter drug behavior - in vitro drug discovery for tumor metabolism has had poor translation in clinical trials, in part due to compensatory functions of the tumor microenvironment.12
Animal models can be used to evaluate the impact of fasting, circadian or behavioral states (stress, tiredness, etc.) on drug response. Figure 2 shows a study conducted by Absorption Systems on the impact of fasting on bioavailability of a target compound in male beagle dogs. The test product (a nanocrystalline suspension) improved bioavailability over the reference product, and lowered the impact of feeding state.
Ocular Delivery Systems
The eye is a common site of pathology that presents unique challenges with regards to drug delivery. Ocular pathologies can occur at different sites in the eye and delivery systems must be developed to optimize bioavailability at the local sites.13 The two most common target sites are anterior segment (outer eye conditions: cataract, corneal wound, and dry-eye disease) and posterior segment (back-of-eye conditions: age-related macular degeneration and diabetic retinopathy). These sites have different barriers to bioavailability that can severely impair the effectiveness of treatment. Recently, novel ocular drug delivery systems have been established to overcome many of these issues.
Anterior segment disorders are typically treated with topical application of therapeutic agents (drops, gel suspensions). These face both static (e.g. corneal epithelium, blood aqueous barrier) and fluid (e.g. tear dilution, blinking) barriers that limit bioavailability. Thus, mechanisms for increasing precorneal residence of drugs to offer sustained release are required. Cyclodextran nanoparticles are cyclic oligosaccharides that form complexes with hydrophobic drugs to enhance corneal residency and reduce inflammation.5,14 High residency-slow release properties of nanoparticles can also reduce dosing frequency.15
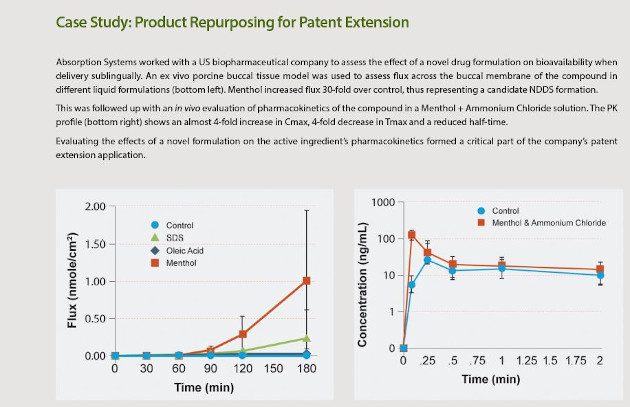
Back-of-eye conditions normally require drug injection to the target site. Intravitreal injection of anti-VEGF drugs to target choroidal neovascularization has been a fruitful area of development since the first VEGF aptamer (Macugen) was FDA approved in 2004.16 Whilst effective, injection methods are still invasive and require high frequency dosing regimens. Ocular devices are providing alternative methods for delivering drugs to the posterior segment of the eye, such as intravitreal implants composed of biodegradable materials. The Durasert™ technology system was used to produce the first intravitreal delivery system for an antiviral drug (ganciclovir) in the treatment of cytomegalovirus (CMV) retinitis.13
Conclusion
Novel drug delivery systems offer promising opportunities for enhancing therapeutic effectiveness of pharmaceutical products whilst benefitting from significant economic and regulatory advantages over new drug compounds. Preclinical models are critical in guiding the development and selection of suitable delivery systems. Understanding the benefits and limitations of in vitro, ex vivo and in vivo models in evaluating these systems is vital.
References
- Tiwari, G., et al., Drug delivery systems: An updated review. Int J Pharm Investig, 2012. 2(1): p. 2-11.
- Services, U.S.D.o.H.a.H. and F.a.D. Administration, Determining Whether to Submit an ANDA or a 505(b)(2) Application, C.f.D.E.a.R. (CDER), Editor. 2017.
- Watanabe, J.H., T. McInnis, and J.D. Hirsch, Cost of Prescription Drug-Related Morbidity and Mortality. Ann Pharmacother, 2018. 52(9): p. 829-837.
- Alten, R., et al., Examining patient preferences in the treatment of rheumatoid arthritis using a discrete-choice approach. Patient Prefer Adherence, 2016. 10: p. 2217-2228.
- Patra, J.K., et al., Nano based drug delivery systems: recent developments and future prospects. J Nanobiotechnology, 2018. 16(1): p. 71.
- Bardal, S., J. Waechter, and D. Martin, Pharmacokinetics, in Applied Pharmacology. 2011, Saunders: Applied Pharmacology.
- Aungst, B.J., Optimizing Oral Bioavailability in Drug Discovery: An Overview of Design and Testing Strategies and Formulation Options. J Pharm Sci, 2017. 106(4): p. 921-929.
- Sambuy, Y., et al., The Caco-2 cell line as a model of the intestinal barrier: in£uence of cell and culture-related factors on Caco-2 cell functional characteristics. Cell Biology and Toxicology, 2005.
- Systems, A. Evaluation of viscosity effects on drug dissolution and permeation across Caco-2 monolayers. Available from: https://www.absorption.com/kc/evaluation-viscosityeffects-drug-dissolution-permeation-across-caco-2-monolayers-using-vitro-dissolutionabsorption-systems-idas/.
- Nunes, R., C. Silva, and L. Chaves, Tissue-based in vitro and ex vivo models for intestinal permeability studies, in Concepts and Models for Drug Permeability Studies. 2016. p. 203-236.
- Brake, K., et al., In vivo Studies for Drug Development via Oral Delivery: Challenges, Animal Models and Techniques. Pharmaceutica Analytica Acta, 2017. 08(09).
- Amoedo, N.D., E. Obre, and R. Rossignol, Drug discovery strategies in the field of tumor energy metabolism: Limitations by metabolic flexibility and metabolic resistance to chemotherapy. Biochim Biophys Acta Bioenerg, 2017. 1858(8): p. 674-685.
- Gote, V., et al., Ocular Drug Delivery: Present Innovations and Future Challenges. J Pharmacol Exp Ther, 2019. 370(3): p. 602-624.
- Lakkakula, J. and R.M. Krause, A vision for cyclodextrin nanoparticles in drug delivery systems and pharmaceutical applications. Nanomedicine, 2014.
- Saari, K.M., et al., Comparison of topical 0.7% dexamethasone-cyclodextrin with 0.1% dexamethasone sodium phosphate for postcataract inflammation. Graefes Arch Clin Exp Ophthalmol, 2006. 244(5): p. 620-6.
- Administration, U.S.F.D., Drug Approval Package: Macugen (Pegaptanib Sodium) Injection. 2004.