The pharmaceutical safety assessment market is experiencing exponential growth in the use of non-animal technologies (NATs) driven by a combination of regulatory, political and social factors as well as advances in science and technology. This advent of new approaches to toxicology and safety assessment marks an exciting opportunity to enhance not only drug development, but also the drug discovery and candidate selection processes.
There are multiple drivers fueling the shift from animal-based technologies to NATs (see Figure 1). One of the major drivers in Europe has been the principle of the 3Rs (replace, reduce, or refine the use of animals for scientific purposes) becoming enshrined in national and international legislation regulating the use of animals in scientific procedures. However, it is the global advances in biology and biotechnology that have revolutionized the use of non-animal technologies in compound development and safety assessment, with scientists developing new in silico and in vitro methods. Today, scientists are using in vitro testing in a coordinated manner with in vivo approaches to enhance drug discovery and development, as well as to achieve the goals of reducing, refining and replacing animals in testing (when regulatory feasible).
Figure 1. Drivers of changeThe ‘New’ Toxicology
In 2007, the US National Academy of Sciences (NAS) published its vision of a “new” toxicology that would leverage advances in biotechnology in order to transform toxicity testing. As NAS put it, “Advances in toxicogenomics, bioinformatics, systems biology, epigenetics, and computational toxicology could transform toxicity testing from a system based on whole-animal testing to one founded primarily on in vitro methods that evaluate changes in biologic processes using cells, cell lines, or cellular components, preferably of human origin.”
What this Means for R&D and its Current Impact
Behind much of the “new” toxicology is a more structured, scientific view of the way cellular systems connect and communicate through various networks. These networks involve complex biochemical interactions between genes, proteins, and small molecules that maintain normal cellular function, control intercellular communication and allow cells to adapt to environmental changes. From a toxicological perspective, this knowledge can be used to map adverse outcome pathways (AOPs), in which the initial interaction of a chemical entity with a biological target (the molecular initiating event) can be identified, together with intermediate key events and the adverse effects in the tissue, organ or whole animal. Such mapping can even extend to a population. The use of AOPs is enabling a shift from in vivo to in vitro and in silico testing; although often a number of in vitro or in silico tests are required to fully replace a single in vivo assay.
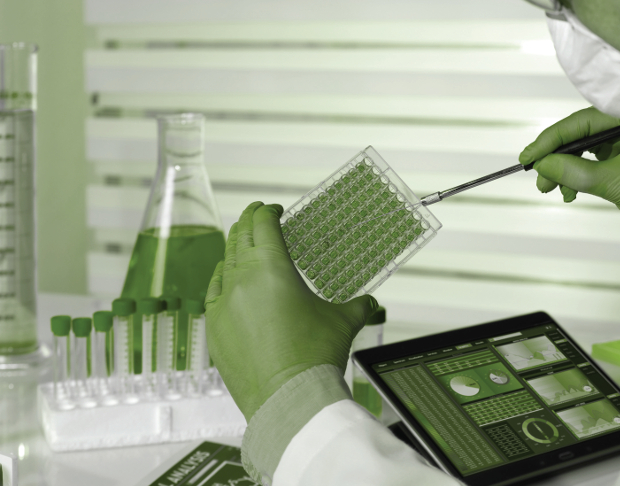
A prime example of NATs replacing in vivo tests is in the assessment of skin sensitization potential. The Local Lymph Node Assay (LLNA), which required the use of mice, was the most commonly used method of testing for skin sensitization. The replacement of the LLNA with a single test was not possible because skin sensitization is multi-factorial. Instead, three alternative assays have now been validated (with further assays still under evaluation (see Table 1) with data generated from these new methods and in silico evaluation used to supply weight-ofevidence (WoE) assessments of an entity’s skin sensitization potential.
Table 1. Example of NATs in skin sensitization testing (status published January 2016)3,4
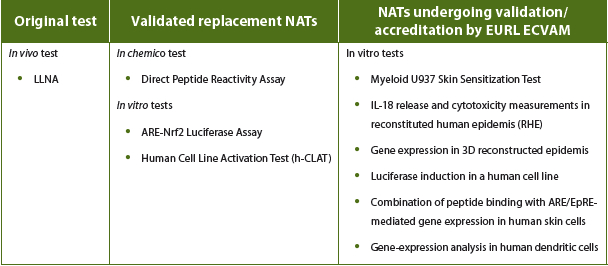
Any new in vitro assays needs to be validated against an in vivo model before authorities can recognize them. In Europe, this recognition is moderated by the EURL ECVAM (European Union Reference Laboratory for Alternatives to Animal Testing), who work with EU-NETVAL (European Union Network of Laboratories for the Validation of Alternative Methods), a network of organizations from across the EU accredited for running validation studies that assess the reliability and relevance of alternative non-animal methods. For each new test, EU-NETVAL validates the new test and EURL ECVAM issues guidance on how and where it can be used. There are 38 accredited labs in this network.
Dynamic 3D in vitro Models
Scientists have been able to grow static 2D and 3D human cell cultures for some time, but these lack the dynamic aspects of the physiological microenvironments created by tissue-to-tissue interactions and the mechanical changes that occur within an organ. One possible solution is the development of organs-on-chips – the chips being microfluidic devices engineered to replicate the in vivo microenvironment. These dynamic 3D or chip models combine the robust, reproducible, easyto- analyze and high-throughput capacity of in vitro technologies with the more physiologically relevant environment achieved by bioengineering the geometrical, mechanical and biochemical factors of the in vivo environment into an in vitro system. Much of the progress in this area is supported by developments in 3D bioprinting and microfluidics (the manipulation of tiny amounts of fluids in channels 10–100 micrometers in diameter). 3D printing creates the structures on which the cells are grown, while microfluidics precisely controls fluid flow within the structure in order to provide nutrients and apply biomechanical stress to the cultured cells. Examples of how these techniques can be used include:
- Toxicity assessments: high content gene quantification assays, ATP content assays, structural cardiotoxicity (and hypertrophy using 3D spheroid models), and structural hepatotoxicity
- Efficacy: testing for drug discovery, target validation (e.g. siRNA knock down), antibody penetration studies and immunomodulatory antibody testing
- Metabolism: metabolism studies
- Drug transport (effects of drugs on tissue efflux and uptake transporters) and mechanisms of idiosyncratic DILI
Microfluidics also plays a pivotal role in the development of hanging drop culture systems - an alternative to the in vitro microstructure approach. These hanging drops, also known as multicellular spheroids or spherical microtissues (MTs), are scaffold-free clusters comprising many cell types that have tissue-like phenotype and function. By connecting hanging drops in arrays, these systems can be developed into pseudoorgans similar to organs-on-a-chip.
Is it All in the Cell Type?
Many of the techniques discussed above enable the maintenance of metabolically competent human tissue in culture for up to 28 days. This provides a time window that scientists can exploit in order to run appropriate toxicology and even biomarker or efficacy tests. It is predicted that in the future there will be an increase in the use of induced pluripotent stem cells, which will allow scientists to program the growth of whatever tissue they need - healthy or diseased - for either therapeutic purposes, disease modeling or toxicology/de-risking studies. We are also likely to reach the stage where scientists can order any organ-on-a-chip they want, complete with whatever genetically based disease they are interested in. This might be achieved using CRISPR or other gene editing technologies to knock out individual genes, especially for monogenic diseases such as cystic fibrosis.
NAT: in silico Modeling
Alongside the development of new in vitro assays, there has also been a huge increase in the use of in silico systems. Advances in the use of Informatics and Quantitative Structure-Activity Relationship (QSAR) modeling now enables analysis of very large datasets - comprising thousands of diverse molecular structures and biological data - using a wide variety of statistical and machine-learning techniques. This analysis provides opportunities for lead optimization through early safety assessment of potential new drugs based on comparisons with other similar chemical structures.
Leveraging These New Approaches Throughout the Whole Drug Discovery Program
Although this article started with a focus on technologies that replace existing in vivo toxicology assays, it is clear that the techniques described could also have applications in drug discovery, including candidate selection and efficacy assays.
Forward-looking CROs are focusing their creativity on delivering novel ideas and in vitro approaches. For example, at Envigo, we set up a new scientific and technical advisory group (STAG) to think creatively about how to develop assays or technologies that answer the big questions and challenges in the pre-clinical R&D (see Figure 2). We are also currently involved in a NATs’ CRACK-IT project that will measure the ability of the TruLarv model (wax moth larva) to correlate with the acute toxicity of compounds in mammals (published LD50 measurements) and to assess whether prediction of toxicity using this model is more accurate than the in vitro OECD 129 cytotoxicity method.
Figure 2. Envigo is an accredited EU-NETVAL memberConclusions
The use of in silico and in vitro technologies in “new” toxicology testing has grown rapidly in recent years. Their use will expand further in the future, with new technologies enhancing drug selection, efficacy testing and toxicology assessment. Developing an integrated approach is the key to capitalizing on market opportunities, making the most of expertise from different disciplines, and thinking creatively and collaboratively about technological solutions to the big challenges that may be faced.
* The original source of the figures and tables is the Envigo White Paper: “Seizing new opportunities in non-animal technologies – an evolving safety testing market”.